
The Prime Wall™
The BamCore Prime Wall™ is a pioneering, carbon-negative framing system ideal for low-rise commercial and residential structures up to five stories
This patented bamboo/Eucalyptus/wood hybrid system seamlessly integrates into all architectural designs and reduces thermal bridges by up to 90% for enhanced energy efficiency. Our innovative Load Optimized Biogenic Industrialized Construction (LOBIC) and 3D Building Information Modeling (BIM) technologies facilitate waste reduction, increase productivity, and lower project time and labor needs without cranes.
​
Delivered as a kit of parts, the Prime Wall panels arrive at the job site as palletized, sequentially numbered units. Each element, including doors, windows, outlets, and access panels, are precision-cut to millimeter accuracy at our factory, eliminating onsite cutting, reducing waste, and maximizing efficiency. Each panel has a Mechanical Engineering Plumbing (MEP) map and nail pattern for a streamlined installation process that increases productivity.
​
Outperforming conventional 2 x 6 framing with twice the strength, BamCore panels sequester more atmospheric carbon than is emitted throughout its harvest, manufacture, and construction life cycle stages. By opting for the Prime Wall, you can reap the benefits of cost, time, and labor savings while swiftly aligning with ESG goals.
​
Please see our Technical Evaluation Report and Typical Details for more information


Stronger = Safer
The Prime Wall™ carries twice the load of 2 x 6 and can stop a small-caliber bullet
​
The BamCore Prime Wall System stands out for its exceptional resilience, achieving Level I protection under the rigorous ballistic resistance guidelines of the National Institute of Justice (NIJ-STD-0108.01 ballistics). This innovative system can resist the impact of .38 Special and .22 long rifle high-velocity rounds when shot from a distance of 16 feet or more, offering substantial protection against a range of handguns.
​
In tests conducted on the core Prime Wall System, which comprises two parallel, load-bearing panels, the system's strength was on full display. Remarkably, these tests were executed without any exterior finish cladding, underlining BamCore's commitment to resilient, high-performance building solutions.
​
In a separate comparison test against a conventional stud-built wall (2 x 6 with studs 12" on center), the BamCore Prime Wall demonstrated superior strength and durability. A 2 x 4 projectile fired at 55 mph barely dented the Prime Wall, while the same projectile easily penetrated the conventional wall. When the speed was upped to 62 mph, the projectile again breached the conventional wall but was stopped by the Prime Wall's outer layer. This exceptional resilience is proof of the Prime Wall's superior construction and design.


ASTM E72 Assembly Vertical Load

Energy Efficient
Revolutionizing the framing factor for superior thermal performance that saves you money year after year
​
The BamCore Prime Wall™ is a nearly hollow wall comprised of two parallel runs - inside and outside - designed to be filled uniformly with any blown-in insulation. In conventional framing, wood studs, posts, or headers that span the wall cavity create a thermal bridge that displaces insulation and undermines thermal performance. Energy analysts use a "framing factor" to calculate the effect of stud-based thermal bridging and estimate how much of the wall is thermally bridged by the wood elements. In a survey of conventional practices in California, the average framing factor was 27%. Today, seismic, wind, and snow loads have driven framing factors up to 30-40% in many locations.
​
The BamCore Prime Wall challenges this paradigm. The estimated framing factor for a standard single-family home using the BamCore system is a mere 6.5%. This results in BamCore exterior walls providing a significantly superior effective R-value from insulation, contributing to an overall impressive "U" value. Thus, it underlines the promise of outstanding thermal performance and heightened energy efficiency.
​
CA Title 24 Compliant in all 16 Climate Zones without toxic exterior rigid foam insulation
​
Recognizing that building operations are a significant source of greenhouse gas emissions, jurisdictions like California have introduced minimum energy efficiency standards in their building codes as a critical measure to combat climate change. The mandatory energy assessment, also known as the Title 24 analysis, is a crucial component of the permitting process for new buildings in California.
​
However, the quest for reduced operational emissions often requires using materials with high-embodied carbon, which inadvertently accelerates climate change. Addressing this paradox, BamCore's Prime Wall System presents a sustainable solution that effectively reduces both operating and upfront greenhouse gas emissions. By addressing both operational and embodied carbon, this innovation is a significant step towards decarbonizing the built environment.
​
For more information, see:


ASTM C1363 Assembly Thermal Resistance

Quieter
Bid farewell to noise pollution and rest easy. The Prime Wall™ achieves an impressive OITC rating of 42, surpassing the 32 of conventional 2 x 6 stud walls
​
The BamCore Prime Wall System improves acoustic comfort in buildings by significantly reducing sound transmission through exterior and interior walls. The Prime Wall system's remarkable acoustic performance is based on a combination of material choice and design that eliminates cavity framing. It effectively reduces sound penetration, as measured by Outside-Inside Transmission Class (OITC) ratings for exterior walls and Sound Transmission Class (STC) ratings for common interior walls. This is particularly important in an era when sound pollution from outdoor sources and across common walls inside commercial or multifamily buildings is a significant concern to owners and occupants.
​
For exterior noises, which usually involve low-frequency sounds, the BamCore exterior Prime Wall boasts an OITC rating of 42, significantly higher than the 32 of a conventional 2×6 stud wall. Regarding interior sounds, primarily high-frequency noises, the BamCore interior Prime Wall clad with a single layer of gypsum achieves an impressive STC rating of 51, surpassing building code requirements. This rating can be improved to 55 by adding a second layer of gypsum.
​
The BamCore Prime Wall System demonstrates that sound attenuation in construction need not be a complex or expensive endeavor but can be achieved with simplicity and effectiveness.


Greener = Healthier
The Prime Wall™ eliminates toxic materials for improved air quality
BamCore prioritizes sustainability, sourcing bamboo mainly from sustainably managed suppliers in developing nations and supporting local economies without resorting to harmful clear-cutting practices.
We extend this commitment to our eco-conscious manufacturing processes, including using carefully chosen adhesives for panel and lumber lamination to reduce embodied carbon and eliminate toxic off-gassing.
​
Our Gen 1 wall panels were subjected to hygrothermal analysis, studying thermal, wind, and humidity interactions to estimate mold growth potential. A Mold Growth Index (MGI) simulation by Passiv Science across 12 cities demonstrated that the BamCore Prime Wall consistently registered a low mold risk with an MGI below 2. This superior thermal performance and reduced mold risk make the Prime Wall an advantageous choice for building envelopes, especially in colder climates. Testing on our Gen 3 Prime Wall is underway.
​
For more information, see:
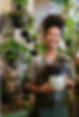
_edited.png)
Installation Speed
Build in half the time, with half the crew, without cranes
​
BamCore's Generation 3 Prime Walls simplify construction, saving time and labor. Using a pneumatic nail gun, a team of three apprentice laborers can assemble 2,800 sqft in four hours. This resource-efficient approach helps ensure your project progresses seamlessly and potentially ahead of schedule.
​
Delivered sequentially, the Prime Wall panels arrive with millimeter precision cutouts for doors, windows, and access panels, eliminating most on-site cutting. Each panel has a MEPI map and nail pattern to streamline installation, increase productivity, and minimize waste.
​
Panels attach easily to the conventional wood top and bottom plates. The panels and BamCore’s web-accessible 3D plans make it easy for the trades to install electrical, plumbing, insulation, and HVAC components.
​
After the rough construction phase, finishing tasks become significantly easier. Due to the rigidity of the entire wall panel, there is no need to search for studs when mounting cabinetry, mirrors, or other heavy items. Plus, the exceptionally flat panel surface and the pre-applied MDO paper on interior panels simplify preparation for painting and scribing tasks.
Mass Timber Bamboo™ (MTB)
Carbon-negative mid- to high-rise materials
(In development)


The Future
BamCore's Mass Timber Bamboo™ (MTB) is set to revolutionize the construction industry, reducing embodied carbon by a staggering 50% compared to conventional steel and concrete used in warehouse construction. This innovative material drastically minimizes embodied carbon at the whole building level, significantly outperforming the average Cross Laminated Timber (CLT).
​
MTB delivers substantial improvements over traditional CLT in multiple ways:
-
It provides up to 98% greater stiffness when compared to the same thickness.
-
It can be up to 27% thinner while maintaining the same stiffness, offering efficiency in space utilization.
-
It is lighter by up to 17%, providing the same stiffness and contributing to ease of transport and installation.
​
When considering the overall embodied carbon of a building, MTB offers remarkable benefits:
-
It can reduce embodied carbon by up to 25% in warehouse constructions.
-
In the context of mid-rise buildings, MTB can cut embodied carbon by up to 20%.
​
In summary, the transformative potential of BamCore's Mass Timber Bamboo is clear. It enhances structural performance and contributes significantly to our collective endeavor to minimize the built environment's carbon footprint.
AI Generated Rendering